Cataloguing Strategic Innovations
The Evolution, Growth, and Status of Hyper-automation: A Pathway to Efficient Digital Transformation
Discover how hyper-automation, the fusion of artificial intelligence, machine learning, and robotic process automation, is transforming organizations across industries. Explore its evolution, benefits, challenges, and the promising future it holds.
In today's rapidly evolving digital landscape, organizations are constantly seeking innovative ways to improve operational efficiency, streamline processes, and enhance productivity. One such transformative approach that has gained significant traction is hyper-automation. Combining artificial intelligence, machine learning, robotic process automation, and intelligent business process management, hyper-automation is revolutionizing the way businesses automate complex tasks and workflows. In this article, we will explore the evolution, growth, current status, adaptation approach, advantages, difficulties, product landscape, and the promising future of hyper-automation.
Hyper-automation is an advanced approach to automation that combines various technologies, including artificial intelligence (AI), machine learning (ML), robotic process automation (RPA), natural language processing (NLP), and intelligent business process management (iBPM). It aims to automate and optimize complex business processes, resulting in increased efficiency, productivity, and agility.
The concept of hyper-automation goes beyond traditional automation, which typically focuses on repetitive and rule-based tasks. Hyper-automation seeks to automate end-to-end processes, encompassing both manual and digital tasks, and involves the integration of multiple technologies to achieve seamless automation across an organization.
Here are some key components of hyper-automation:
1. Robotic Process Automation (RPA): RPA involves the use of software robots or "bots" to mimic human actions and perform rule-based tasks on computer systems. RPA can automate tasks such as data entry, data extraction, and report generation.
2. Artificial Intelligence (AI) and Machine Learning (ML): AI and ML technologies are utilized to enable intelligent automation. They allow systems to learn from data, make decisions, and perform tasks that traditionally require human intelligence. AI-powered algorithms can be used for data analysis, natural language understanding, image recognition, and predictive modeling.
3. Natural Language Processing (NLP): NLP enables computers to understand and interpret human language. It processes and analyzes unstructured data, such as customer emails, social media posts, or documents. NLP can be leveraged to automate tasks like sentiment analysis, chatbots, and language translation.
4. Intelligent Business Process Management (iBPM): iBPM combines business process management (BPM) with AI capabilities. It helps organizations model, optimise, and automate complex business processes. iBPM platforms often incorporate process mining, business rules management, workflow automation, and decision management.
5. Process Discovery and Mining: Hyperautomation involves identifying and analyzing existing business processes through process discovery and mining techniques. By monitoring user interactions with software systems, organizations can gain insights into how tasks are performed, identify bottlenecks, and discover automation opportunities.
6. Integration and Orchestration: Hyperautomation requires integrating and orchestrating various systems, applications, and technologies. This ensures smooth data flow and communication between different components, allowing end-to-end automation across the organization.
The benefits of hyper-automation include improved operational efficiency, reduced costs, increased accuracy, faster processing times, enhanced customer experiences, and the ability to scale and adapt to changing business needs. Organizations can streamline operations and focus on higher-value tasks by automating complex processes and augmenting human capabilities with AI.
Evolution and Growth: The concept of hyper-automation emerged as a natural progression from traditional automation practices. Initially, organizations focused on automating repetitive, rule-based tasks using robotic process automation (RPA). However, as technology advanced, the need for intelligent automation became evident. The integration of AI, ML, and NLP into RPA systems enabled organizations to automate more complex processes and handle unstructured data. This fusion of technologies gave rise to hyper-automation, which aims to automate end-to-end processes and optimize business operations.
In recent years, hyper-automation has experienced remarkable growth across industries. According to Gartner's Hyperautomation Hype Cycle, it has transitioned from the "Innovation Trigger" phase to the "Peak of Inflated Expectations," indicating the growing interest and adoption. Organizations are recognizing the potential of hyper-automation to drive digital transformation and gain a competitive edge. The market has witnessed the emergence of various solution providers offering hyper-automation platforms and tools to facilitate its implementation.
Adaptation Approach: Implementing hyper-automation requires a systematic approach that involves understanding existing processes, identifying automation opportunities, and leveraging appropriate technologies. The adaptation approach typically includes the following steps:
1. Process Discovery: Organizations analyze their business processes to identify areas for automation. Process mining and analysis tools help in gaining insights into process flows, bottlenecks, and potential areas of improvement.
2. Automation Design: Once the processes are identified, organizations design automation workflows and select the appropriate technologies based on the complexity and requirements of each task. This includes leveraging RPA, AI, ML, NLP, and iBPM tools to automate both digital and manual tasks.
3. Integration and Orchestration: Successful hyper-automation implementation requires integrating various systems, applications, and technologies. Seamless data flow and communication ensure a cohesive automation ecosystem. Integration platforms facilitate the smooth functioning of automated workflows.
4. Monitoring and Optimization: Continuous monitoring and analysis of automated processes help organizations identify areas for further optimization. Feedback loops and machine learning algorithms enable systems to learn and improve over time.
Advantages and Difficulties: Hyperautomation offers numerous advantages to organizations embarking on their digital transformation journey:
1. Increased Efficiency and Productivity: By automating complex processes, organizations can significantly improve efficiency, reduce errors, and boost productivity. Automated tasks are performed faster and more accurately, allowing employees to focus on strategic initiatives.
2. Enhanced Customer Experience: Hyperautomation enables organizations to streamline customer-facing processes, leading to improved response times, personalized interactions, and better service quality. Chatbots, sentiment analysis, and language translation enhance customer satisfaction.
3. Cost Reduction: By automating tasks traditionally performed by humans, organizations can achieve cost savings. Reduced manual efforts, increased throughput, and improved accuracy contribute to overall cost optimization.
4. Agility and Scalability: Hyperautomation enables organizations to respond quickly to market changes, adapt to evolving customer demands, and scale their operations. Flexible workflows and dynamic resource allocation support business growth.
While hyper-automation brings significant benefits, certain challenges must be addressed:
1. Complexity and Integration: Orchestrating multiple technologies and integrating various systems can be complex. Organizations need expertise and resources to handle the technical intricacies and ensure smooth interoperability.
2. Change Management: Implementing hyper-automation requires cultural and organizational change. Employees must adapt to new roles, reskill, and embrace the technology-driven workplace. Effective change management strategies are crucial for successful adoption.
3. Data Privacy and Security: As hyper-automation involves handling large volumes of data, organizations must ensure robust security measures and comply with data privacy regulations. Safeguarding sensitive information and protecting against cyber threats is paramount.
Products and Comparison: The hyper-automation market offers a diverse range of products and platforms. Some of the notable solutions include:
1. UiPath: A leading RPA provider, UiPath offers an end-to-end hyper-automation platform that combines RPA, AI, and ML capabilities. It supports intelligent automation, process mining, and easy integration with other systems.
2. Automation Anywhere: Automation Anywhere provides an AI-powered RPA platform that enables organizations to automate complex processes, integrate AI technologies, and optimize workflows through its intelligent automation capabilities.
3. Blue Prism: Blue Prism offers an enterprise-grade RPA platform with cognitive capabilities. It supports integration with AI technologies, process analytics, and scalable automation.
Hyperautomation has the potential to benefit organizations across various industries and sectors. Here are some examples of the types of organizations that can leverage hyper-automation to drive efficiency, productivity, and digital transformation:
- Financial Institutions: Banks, insurance companies, and financial service providers can benefit from hyper-automation to streamline complex processes such as customer onboarding, loan origination, claims processing, fraud detection, and compliance. Hyperautomation enables faster and more accurate data analysis, risk assessment, and regulatory reporting.
- Healthcare Providers: Hospitals, clinics, and healthcare organizations can utilize hyper-automation to optimize patient care, medical records management, appointment scheduling, billing, and insurance claims processing. Automation can help reduce administrative burdens, improve accuracy in medical data handling, and enhance patient experience through personalized care.
- Manufacturing and Supply Chain: Manufacturing companies can automate production processes, inventory management, supply chain coordination, and quality control using hyper-automation. Intelligent automation facilitates real-time data analysis, demand forecasting, inventory optimization, and efficient order fulfillment.
- Retail and E-commerce: Retailers and e-commerce businesses can leverage hyper-automation to automate inventory management, order processing, logistics, customer support, and personalized marketing campaigns. Automation can improve inventory accuracy, order fulfillment speed, customer service responsiveness, and targeted marketing strategies.
- Human Resources: HR departments can benefit from hyper-automation in tasks such as employee onboarding, payroll management, leave and attendance tracking, performance evaluations, and talent acquisition. Automation streamlines administrative processes reduces manual errors, and enhances employee engagement.
- Customer Service and Support: Organizations dealing with customer service and support, such as call centers, can utilize hyper-automation to enhance customer experiences. Chatbots and virtual assistants powered by natural language processing can provide instant support, handle routine inquiries, and escalate complex issues to human agents.
- Energy and Utilities: Hyperautomation can help energy and utility companies optimize energy distribution, metering, billing, and customer service. Automated systems can monitor energy consumption, detect anomalies, and improve energy efficiency through predictive analytics and intelligent demand management.
- Government and Public Sector: Government agencies and public sector organizations can leverage hyper-automation to enhance citizen services, automate administrative processes, facilitate document management, and improve data analysis for policy-making. Automation can streamline permit processing, license renewals, tax filings, and citizen engagement.
- Transportation and Logistics: Hyperautomation can optimize transportation and logistics operations by automating route planning, shipment tracking, fleet management, and supply chain visibility. Intelligent automation enables real-time data integration, analytics, and predictive maintenance to improve efficiency and reduce costs.
It's important to note that the potential benefits of hyper-automation extend beyond these examples, and organizations in various other industries can also harness its power to optimize their processes, improve decision-making, and drive business growth. The specific applications and benefits will vary based on the unique needs and challenges of each organization. Top of Form
Bottom of Form
Future Outlook: The future of hyper-automation appears promising, with continued growth and innovation. As technologies mature and AI algorithms become more sophisticated, hyper-automation will become increasingly accessible and powerful. Advancements in natural language understanding, computer vision, and predictive analytics will further enhance its capabilities.
Furthermore, hyper-automation will enable the rise of the "Digital Workforce," combining human and digital workers to collaborate seamlessly. Organizations will focus on augmenting human capabilities with AI-driven automation, creating a symbiotic relationship to drive efficiency and innovation.
Conclusion: Hyperautomation is reshaping the way organizations approach automation and digital transformation. With its integration of AI, ML, RPA, NLP, and iBPM, it offers a comprehensive solution for end-to-end process automation. While challenges exist, the advantages of increased efficiency, cost reduction, enhanced customer experience, and agility make hyper-automation a compelling choice for organizations seeking competitive advantage. As technology evolves and matures, hyper-automation will continue to revolutionize industries, empowering organizations to achieve new levels of productivity and success.
Blockchain Technology: A General Purpose Technology for the Digital Age
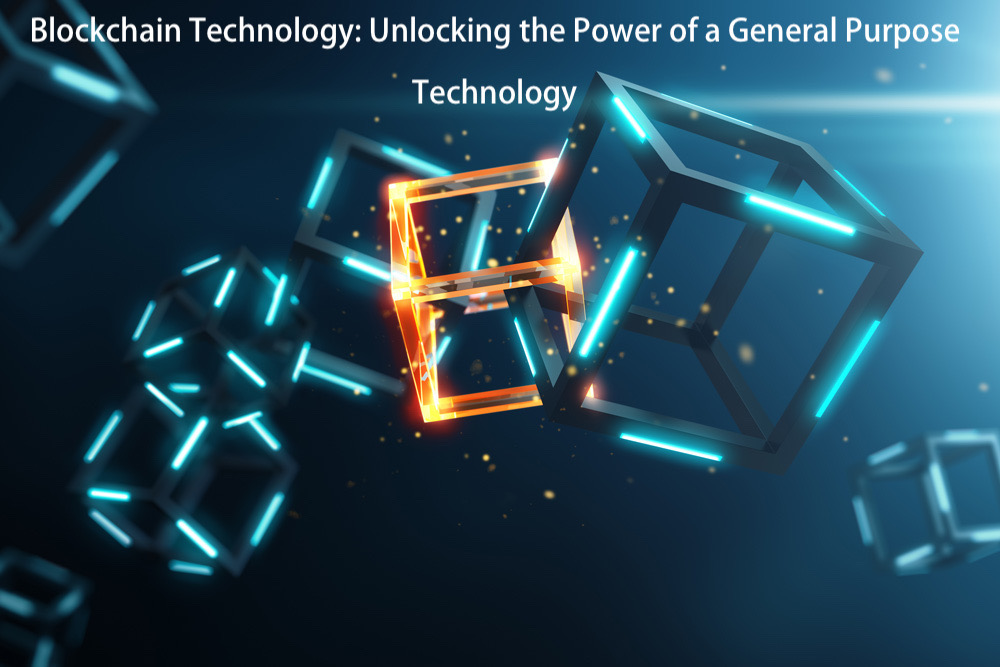
Unlocking the Power of a General Purpose Technology
Discover the immense potential of blockchain technology as a general-purpose technology (GPT) that is reshaping industries and driving innovation. Explore the key characteristics that classify blockchain as a GPT and understand its transformative impact on various sectors.
Blockchain technology has emerged as a transformative force in the digital realm, revolutionizing various industries and challenging traditional systems. What sets blockchain apart is its classification as a general purpose technology (GPT), a term used to describe technologies that have broad and profound impacts across multiple sectors. This article will explore why blockchain is considered a general-purpose technology and delve into its remarkable potential and significance.
Blockchain is a decentralized and distributed ledger technology that allows multiple parties to maintain a shared and immutable record of transactions or data securely and transparently. It gained prominence with the introduction of Bitcoin in 2009, but its applications extend far beyond cryptocurrencies.
The technology behind blockchain revolves around a network of computers, known as nodes, that collectively validate and store transactions or data. Each transaction or data entry is bundled into a block, which is linked to the previous blocks in chronological order, forming a chain of blocks, hence the name "blockchain." This chaining of blocks ensures the integrity and immutability of the recorded information.
The evolution of blockchain technology can be divided into three generations:
1. First-generation blockchain: Bitcoin introduced the concept of a decentralized, peer-to-peer electronic cash system, establishing the foundation of blockchain technology.
2. Second-generation blockchain: Ethereum, launched in 2015, expanded the capabilities of blockchain by introducing smart contracts. Smart contracts are self-executing contracts with the terms of the agreement directly written into code. They enable the automation of transactions, eliminating the need for intermediaries in various applications.
3. Third-generation blockchain: This phase focuses on scalability, interoperability, and privacy. It aims to address the limitations of previous generations by introducing innovations such as sharding, sidechains, and consensus mechanisms like Proof of Stake (PoS). Projects like Polkadot, Cardano, and Cosmos fall into this category.
The importance of blockchain lies in its ability to provide trust, transparency, security, and efficiency in various sectors. Here are some key aspects that highlight its significance:
1. Trust and transparency: Blockchain removes the need for intermediaries by providing a decentralized and transparent system. Participants can verify transactions or data without relying on a central authority.
2. Security: Blockchain uses cryptographic techniques to secure transactions and prevent unauthorized alterations. The decentralized nature of the network also makes it more resilient to attacks.
3. Efficiency: Blockchain streamlines processes by eliminating intermediaries, reducing costs, and increasing the speed of transactions. It enables peer-to-peer transactions and automated execution of smart contracts.
Blockchain can be classified as a general-purpose technology (GPT) due to its potential to disrupt and transform various industries. GPTs possess several key characteristics:
1. Wide applicability: Blockchain has applications in finance, supply chain management, healthcare, voting systems, intellectual property, energy, and more. Its potential use cases span multiple sectors.
2. Complementary to existing technologies: Blockchain can work in conjunction with other technologies such as the Internet of Things (IoT), artificial intelligence (AI), and cloud computing, enhancing their capabilities and creating new synergies.
3. Evolving and expanding impact: The technology continues to evolve, with ongoing research and development efforts focused on scalability, interoperability, and privacy. As it matures, blockchain is likely to have a profound impact on numerous industries.
Some notable applications of blockchain include:
1. Supply chain management: Blockchain can provide end-to-end traceability and transparency in supply chains, helping track products, verify authenticity, and prevent fraud.
2. Healthcare: Blockchain can enhance the security and privacy of medical records, facilitate interoperability among healthcare providers, and streamline processes like drug supply chain management and clinical trials.
3. Decentralized finance (DeFi): Blockchain enables the creation of decentralized financial applications, including lending platforms, decentralized exchanges, and stablecoins, offering greater accessibility and financial inclusivity.
4. Identity management: Blockchain can empower individuals with self-sovereign identities, giving them control over their personal information and simplifying identity verification processes.
5. Cryptocurrencies and digital assets: Blockchain serves as the underlying technology for cryptocurrencies like Bitcoin and Ethereum, enabling secure and transparent peer-to-peer transactions.
The future of blockchain holds tremendous potential. As the technology matures,
Blockchain operates through a series of key components and processes that work together to ensure the integrity and security of the data stored within it. Here's a simplified explanation of how blockchain works:
1. Distributed Network: Blockchain is a decentralized system consisting of multiple nodes (computers) connected over a network. Each node maintains a copy of the entire blockchain, making it highly resilient and resistant to single points of failure.
2. Blocks: Transactions or data entries are grouped into blocks. A block contains a collection of these records, along with a unique identifier called a "hash" and a reference to the previous block's hash.
3. Hashing: Each block is assigned a unique cryptographic hash, which is a fixed-length alphanumeric string generated using a mathematical algorithm. This hash serves as a digital fingerprint for the block, ensuring its integrity.
4. Chain of Blocks: The blocks are arranged in chronological order, forming a chain. The hash of each block is based on its data and the hash of the previous block, effectively linking the blocks together. This linkage ensures that any tampering with a block would require altering subsequent blocks, making the blockchain resistant to manipulation.
5. Consensus Mechanism: To maintain the integrity of the blockchain, a consensus mechanism is used to agree on the validity of new blocks. The most well-known consensus mechanism is Proof of Work (PoW), where nodes compete to solve a complex mathematical puzzle to validate transactions and add a new block to the chain. Other consensus mechanisms, such as Proof of Stake (PoS) and Delegated Proof of Stake (DPoS), use different methods to achieve consensus.
6. Validation and Verification: Transactions within a block are validated by the participating nodes according to predefined rules or smart contracts. Once verified, the transactions are bundled into a new block and added to the chain.
7. Immutable and Transparent: Once a block is added to the blockchain, it becomes virtually impossible to alter or delete the data within it without the consensus of the majority of the network. This immutability ensures that the transaction history recorded on the blockchain is tamper-proof and transparent.
8. Security: The decentralized nature of blockchain, coupled with cryptographic hashing and consensus mechanisms, makes it highly secure against fraud, tampering, and unauthorized access. Any attempts to alter the data would require a majority of the network's computing power, making it extremely difficult and cost-prohibitive.
By combining these elements, blockchain technology provides a trustless and transparent system where participants can securely store and exchange data without relying on intermediaries or central authorities.
Understanding General Purpose Technology:
General purpose technologies possess unique characteristics that enable them to transform various industries and significantly impact economic and social systems. They typically exhibit the following attributes:
- Wide Applicability: GPTs can be applied across diverse sectors and industries, transcending specific use cases. They provide a foundation for innovation, enabling the development of a wide range of applications and solutions.
- Complementary Nature: GPTs often interact with and enhance other technologies, creating synergies and amplifying their capabilities. They catalyze innovation and facilitate the integration of multiple technological advancements.
- Economic Spillover Effects: GPTs generate positive externalities by driving productivity improvements, fostering economic growth, and facilitating the development of new markets and business models. Their impact extends beyond the sectors directly utilizing the technology.
Blockchain as a General Purpose Technology:
Blockchain technology possesses the fundamental characteristics of a general-purpose technology, which contribute to its versatility, transformative potential, and widespread adoption. Here's why blockchain is considered a GPT:
- Decentralization and Trust: Blockchain's decentralized nature eliminates the need for intermediaries, enabling peer-to-peer interactions with enhanced transparency and trust. It establishes a distributed and immutable ledger where transactions are securely recorded and verified by a network of participants, reducing the reliance on centralized authorities.
- Programmable Smart Contracts: Smart contracts are self-executing agreements embedded within the blockchain. They automate and enforce contract terms, eliminating the need for intermediaries, streamlining processes, and reducing costs. Smart contracts enable the creation of complex decentralized applications (DApps) and facilitate innovative business models.
- Security and Data Integrity: Blockchain technology employs robust cryptographic mechanisms to ensure data integrity, immutability, and tamper resistance. The decentralized consensus mechanism protects against fraud and unauthorized modifications, enhancing the security of digital assets and sensitive information.
- Interoperability and Standards: Blockchain's open architecture and standardized protocols promote interoperability among various blockchain networks and applications. This interoperability facilitates seamless data exchange, collaboration, and the integration of disparate systems, driving innovation and efficiency.
- Tokenization and Digital Assets: Blockchain enables the tokenization of real-world assets, representing them as digital assets on the blockchain. This tokenization opens up new possibilities for fractional ownership, increased liquidity, and the creation of new asset classes, such as digital currencies, non-fungible tokens (NFTs), and security tokens.
Organizations considering deploying blockchain technology should follow a systematic approach to ensure successful implementation and maximize the benefits. Here are some key steps to consider:
1. Identify the Problem: Start by identifying the specific problem or inefficiency that blockchain can address within your organization or industry. Determine if blockchain is the right solution and understand its potential benefits and limitations.
2. Conduct a Feasibility Study: Perform a feasibility study to assess the technical and economic viability of implementing blockchain. Evaluate factors such as scalability, security, regulatory compliance, interoperability, and potential return on investment (ROI).
3. Define Use Case and Requirements: Clearly define the use case for blockchain within your organization. Identify the specific requirements and functionalities needed, such as transaction volume, data privacy, smart contract capabilities, consensus mechanism, and integration with existing systems.
4. Choose the Right Blockchain Platform: Select a suitable blockchain platform that aligns with your use case and requirements. Consider factors such as scalability, consensus mechanism, programming language support, community and ecosystem, and enterprise-grade features. Popular platforms include Ethereum, Hyperledger Fabric, Corda, and EOS.
5. Build or Partner: Decide whether to build the blockchain solution in-house or partner with a specialized blockchain development firm or consortium. Building in-house provides more control but requires significant expertise while partnering can expedite development and deployment.
6. Design the Architecture: Design the blockchain architecture based on your use case and requirements. Determine the network structure, node configuration, data storage mechanisms, smart contract design, and integration points with existing systems or external data sources.
7. Develop and Test: Develop the blockchain solution, including smart contracts, consensus mechanisms, user interfaces, and integration components. Conduct thorough testing to ensure functionality, security, and performance.
8. Deployment and Integration: Deploy the blockchain solution in a production environment and integrate it with existing systems or processes. Ensure proper connectivity, data synchronization, and interoperability with relevant stakeholders.
9. Governance and Network Management: Establish governance mechanisms for managing the blockchain network, including consensus protocol upgrades, security protocols, participant onboarding, and dispute resolution mechanisms.
10. User Adoption and Training: Promote user adoption by providing training and support for stakeholders who will interact with the blockchain solution. Educate users on the benefits, functionalities, and proper usage of the technology.
11. Monitor and Iterate: Continuously monitor the performance and efficiency of the blockchain solution. Collect feedback from users and stakeholders, and iterate the system based on real-world experiences and evolving needs.
12. Collaboration and Ecosystem Engagement: Engage with relevant industry consortia, standards bodies, and regulatory authorities to collaborate, share best practices, and shape the development of blockchain technology within your industry.
It's important to note that blockchain implementation requires careful consideration of technical, regulatory, and business aspects. Organizations should involve cross-functional teams, including IT, legal, operations, and management, to ensure a comprehensive approach throughout the deployment process.
There are several blockchain platforms available, each with its features, design principles, and use cases. Here are some of the prominent blockchain platforms:
1. Ethereum: Ethereum is a widely used blockchain platform that introduced the concept of smart contracts. It supports the development and execution of decentralized applications (DApps) and enables the creation of custom blockchain solutions. Ethereum uses a consensus mechanism called Proof of Stake (PoS), which is being implemented through the Ethereum 2.0 upgrade.
2. Hyperledger Fabric: Hyperledger Fabric is an open-source blockchain platform hosted by the Linux Foundation. It is designed for enterprise use cases and provides a modular architecture, allowing organizations to build private and permissioned blockchain networks. Fabric supports smart contracts written in various programming languages and offers a high level of privacy and scalability.
3. Corda: Corda is another open-source blockchain platform designed specifically for business applications. It focuses on maintaining privacy and data integrity within a network of participants. Corda's unique feature is its ability to facilitate direct transactions between parties, known as "CorDapps," without broadcasting the entire network.
4. Binance Smart Chain (BSC): Binance Smart Chain is a blockchain platform developed by the cryptocurrency exchange Binance. It aims to provide a high-performance and low-cost environment for decentralized applications and token issuance. BSC uses a variant of the Proof of Stake (PoS) consensus mechanism and is compatible with the Ethereum Virtual Machine (EVM).
5. Stellar: Stellar is a blockchain platform focused on facilitating fast and low-cost cross-border transactions. It provides a decentralized network for issuing, transferring, and exchanging digital assets, including cryptocurrencies and fiat currencies. Stellar uses a consensus mechanism called Stellar Consensus Protocol (SCP).
6. Cardano: Cardano is a blockchain platform that emphasizes security, sustainability, and scalability. It uses a research-driven approach and aims to provide a platform for the development of decentralized applications and smart contracts. Cardano's native cryptocurrency is ADA, and it utilizes a Proof of Stake (PoS) consensus mechanism.
7. EOS: EOS is a blockchain platform designed for high-speed and scalable decentralized applications. It utilizes a Delegated Proof of Stake (DPoS) consensus mechanism, where a limited number of elected nodes are responsible for validating transactions. EOS aims to provide a developer-friendly environment for building and deploying DApps.
These are just a few examples of blockchain platforms, and there are many others available, each with its unique features, consensus mechanisms, and target use cases. Organizations should carefully evaluate the platforms based on their specific requirements, scalability needs, privacy considerations, developer ecosystem, and community support before choosing the most suitable one for their blockchain deployment.
Comparing blockchain platforms requires considering various factors such as scalability, consensus mechanism, development flexibility, security, community support, and use case suitability. Here's a high-level comparison of the mentioned platforms:
1. Ethereum:
- Scalability: Ethereum has faced scalability challenges with high transaction fees and network congestion. However, Ethereum 2.0 aims to address these issues with the introduction of PoS and shard chains.
- Consensus Mechanism: Ethereum currently uses Proof of Work (PoW), but it is transitioning to PoS. This transition is expected to improve energy efficiency and reduce the barriers to participation.
- Development Flexibility: Ethereum has a robust ecosystem and supports smart contracts written in Solidity. It has a large developer community and offers extensive tooling and documentation.
- Security: Ethereum has faced security vulnerabilities in the past, mainly due to flaws in smart contracts. However, ongoing audits and best practices help mitigate risks.
- Use Cases: Ethereum is widely used for decentralized finance (DeFi), token issuance, and DApp development.
2. Hyperledger Fabric:
- Scalability: Hyperledger Fabric allows for the creation of private and permissioned networks, providing scalability options suitable for enterprise use cases.
- Consensus Mechanism: Fabric supports pluggable consensus mechanisms, including Practical Byzantine Fault Tolerance (PBFT) and Raft, offering flexibility in choosing the consensus algorithm based on network requirements.
- Development Flexibility: Fabric supports multiple programming languages and provides a modular architecture, allowing developers to design custom solutions with specific components.
- Security: Fabric focuses on privacy and confidentiality, with data isolation between participants and support for private channels. It offers granular access control and robust identity management.
- Use Cases: Hyperledger Fabric is often utilized in supply chain management, trade finance, healthcare, and other enterprise applications where privacy and permission access are critical.
3. Corda:
- Scalability: Corda is designed to scale horizontally by allowing each participant to only store relevant transaction data, ensuring efficiency in large networks.
- Consensus Mechanism: Corda utilizes a unique consensus mechanism based on the Notary service, where transaction validity is verified by mutually trusted parties involved in the transaction.
- Development Flexibility: Corda supports developing CorDapps using Kotlin and offers a comprehensive set of libraries and tools for building distributed applications.
- Security: Corda prioritizes privacy and confidentiality, enabling selective sharing of data between participants through secure channels. It employs cryptographic techniques to protect sensitive information.
- Use Cases: Corda is suitable for use cases involving complex business agreements, financial transactions, supply chain networks, and inter-organizational collaborations.
4. Binance Smart Chain (BSC):
- Scalability: BSC aims to offer higher scalability and lower transaction fees compared to Ethereum, making it appealing for decentralized applications that require fast and cost-effective transactions.
- Consensus Mechanism: BSC uses a variant of the PoS consensus mechanism called Proof of Staked Authority (PoSA), where a set of validators is elected to validate transactions and produce blocks.
- Development Flexibility: BSC is compatible with the Ethereum Virtual Machine (EVM), meaning developers can reuse existing Ethereum smart contracts and tools to build on BSC.
- Security: BSC benefits from the security of the Binance ecosystem and has implemented measures to prevent common smart contract vulnerabilities.
- Use Cases: BSC is often utilized for decentralized finance (DeFi) applications, token swaps, and yield farming.
It's important to note that the suitability of a blockchain platform depends on the specific requirements and use case of the organization. Organizations should thoroughly evaluate these platforms, considering their unique needs, ecosystem support, scalability requirements, regulatory considerations, and development resources before making a decision.
Implementing blockchain technology requires careful planning and adherence to best practices to ensure successful deployment. Here are some key best practices to consider:
1. Clearly Define Objectives: Clearly define the objectives and expected outcomes of implementing blockchain technology. Identify the specific problem or inefficiency that blockchain can address within your organization or industry.
2. Conduct a Feasibility Study: Perform a thorough feasibility study to assess the technical and economic viability of blockchain implementation. Evaluate factors such as scalability, security, regulatory compliance, interoperability, and potential return on investment (ROI).
3. Select the Right Use Case: Choose the appropriate use case for blockchain that aligns with your organization's goals and requirements. Look for use cases where blockchain's characteristics, such as decentralization, immutability, and transparency, provide significant advantages over traditional systems.
4. Determine the Blockchain Type: Determine the type of blockchain that suits your use case—public, private, or hybrid. Public blockchains offer transparency but may have scalability limitations, while private blockchains provide more control but sacrifice some decentralization.
5. Involve Stakeholders: Engage key stakeholders, including executives, users, IT teams, and legal departments, early in the process. Seek their input, address concerns, and ensure alignment throughout the implementation.
6. Design for Scalability and Performance: Consider scalability and performance requirements from the outset. Select a blockchain platform or architecture that can handle the expected transaction volume and user base. Pay attention to network design, consensus mechanisms, and data storage mechanisms.
7. Ensure Data Privacy and Security: Assess and address data privacy and security concerns. Implement appropriate access controls, encryption mechanisms, and user identity management. Ensure compliance with relevant regulations, such as data protection and privacy laws.
8. Integration with Existing Systems: Plan for seamless integration with existing systems and processes. Identify integration points, data flows, and APIs needed to connect the blockchain solution with other applications or databases.
9. Smart Contract Auditing and Security: Conduct thorough audits and security assessments of smart contracts to identify vulnerabilities and potential risks. Follow best practices for smart contract development, including code reviews, testing, and using established security frameworks.
10. User Experience and Adoption: Focus on providing a smooth user experience to encourage adoption. Simplify user interfaces, provide training and support, and communicate the benefits of blockchain technology to users and stakeholders.
11. Continuous Testing and Monitoring: Implement robust testing procedures throughout the development and deployment phases. Perform regular monitoring and auditing of the blockchain network for performance, security, and compliance.
12. Collaborate and Leverage Resources: Engage with blockchain communities, industry consortia, and relevant standards bodies to share knowledge, learn from others, and leverage existing resources. Collaborate with other organizations and industry partners to drive interoperability and standardization.
13. Governance and Updates: Establish a governance framework to manage the blockchain network and address protocol upgrades, consensus changes, and decision-making processes. Stay updated with the latest advancements and developments in the blockchain space.
14. Evaluate and Iterate: Continuously evaluate the effectiveness of the implemented blockchain solution. Gather feedback from users, stakeholders, and technical teams. Identify areas for improvement and iterate the system based on real-world experiences.
Remember, blockchain implementation requires a multidisciplinary approach involving technical, operational, legal, and business considerations. It's essential to have a well-defined strategy, a capable development team, and a clear understanding of the benefits and limitations of blockchain technology.
While blockchain technology offers numerous benefits, it's important to be aware of the potential risks and challenges associated with its implementation. Here are some key risks to consider:
1. Security Vulnerabilities: Although blockchain technology provides strong security features, no system is completely immune to vulnerabilities. Smart contract bugs, coding errors, and weaknesses in the underlying cryptography can expose the blockchain to security breaches and hacking attempts.
2. Regulatory Compliance: Blockchain implementation may face challenges in complying with existing regulations. Depending on the jurisdiction and the nature of the data or transactions being recorded, organizations may need to navigate legal and regulatory frameworks related to data protection, privacy, anti-money laundering (AML), and know-your-customer (KYC) requirements.
3. Scalability and Performance: Traditional blockchain platforms, such as public blockchains like Bitcoin and Ethereum, can face scalability limitations. As more transactions are added to the blockchain, it can lead to increased network congestion and slower transaction processing times. Achieving high throughput and scalability remains a technical challenge for blockchain systems.
4. Governance and Consensus Issues: The decentralized nature of blockchain can introduce governance challenges. Decision-making processes, protocol upgrades, and resolving disputes among network participants require consensus, which can be complex and time-consuming. Lack of consensus or disputes within the network can hinder progress and cause fragmentation.
5. Data Privacy Concerns: While blockchain provides transparency and immutability, ensuring data privacy can be a challenge. Public blockchains store all transaction data publicly, raising concerns about sensitive or confidential information. Private blockchains provide more control, but data access and management need to be carefully designed to protect privacy.
6. Energy Consumption: Some blockchain platforms, especially those using Proof of Work (PoW) consensus mechanisms, consume significant amounts of energy for mining and maintaining the network. This raises environmental concerns and may not be sustainable in the long term. Transitioning to more energy-efficient consensus mechanisms, such as Proof of Stake (PoS), is being explored to address this issue.
7. Interoperability and Standards: Blockchain interoperability, the ability of different blockchains to communicate and exchange data seamlessly, is still a challenge. A lack of standardization and interoperability frameworks can hinder the integration of blockchain solutions with existing systems and limit their potential benefits.
8. Adoption and Usability: Blockchain technology may face adoption challenges due to factors such as complexity, user experience, and resistance to change. The learning curve associated with understanding blockchain concepts and the need for new infrastructure and skillsets can slow down adoption within organizations.
9. Economic and Financial Risks: The volatility of cryptocurrencies, which often underpin blockchain ecosystems, can introduce economic and financial risks. Market fluctuations, regulatory changes, and scams within the cryptocurrency space can impact the stability and reliability of blockchain-based systems.
10. Overhyped Expectations: Blockchain technology has been subject to hype and inflated expectations, leading to unrealistic claims and investments in projects that may not deliver the expected outcomes. It's important to have a clear understanding of the technology's capabilities and limitations to avoid misaligned expectations.
To mitigate these risks, organizations should conduct thorough risk assessments, implement robust security measures, stay informed about regulatory developments, choose appropriate consensus mechanisms, and carefully consider the scalability and privacy requirements of their specific use cases. Collaboration with industry partners, adherence to best practices, and ongoing monitoring and evaluation are crucial for successful blockchain implementation.
Cryptocurrencies, such as Bitcoin and Ethereum, are not inherently unsafe or dangerous. However, there are risks and challenges associated with their use that users should be aware of. Here are some reasons why cryptocurrencies can be perceived as unsafe or dangerous:
1. Price Volatility: Cryptocurrencies are known for their significant price volatility. The value of cryptocurrencies can experience dramatic fluctuations within short periods, leading to potential financial risks for investors and users. Rapid price changes can result in significant gains or losses, making cryptocurrencies risky assets to hold or trade.
2. Lack of Regulation: Cryptocurrencies operate in a relatively unregulated space, which can make them susceptible to fraud, scams, and market manipulation. The absence of a centralized authority overseeing cryptocurrency transactions and exchanges means that users have fewer legal protections and recourse in case of fraudulent activities.
3. Security Vulnerabilities: While blockchain technology, the underlying technology behind cryptocurrencies, provides robust security features, the associated applications and infrastructure may have vulnerabilities. Hacking attempts, phishing attacks, and security breaches targeting cryptocurrency wallets, exchanges, or smart contracts can result in the loss or theft of digital assets.
4. Irreversibility of Transactions: Cryptocurrency transactions, once confirmed on the blockchain, are typically irreversible. In cases of accidental or fraudulent transactions, recovering funds or reversing transactions can be extremely challenging or impossible, leading to potential financial losses.
5. Lack of Consumer Protection: Traditional financial systems often have consumer protection mechanisms in place, such as chargebacks and dispute resolution processes. However, cryptocurrencies typically lack such safeguards. If a user sends funds to the wrong address or falls victim to a scam, recovering the funds can be difficult or impossible.
6. Regulatory and Legal Risks: The regulatory environment surrounding cryptocurrencies is evolving and varies across jurisdictions. Unclear or inconsistent regulations can create legal risks for cryptocurrency users and businesses, such as potential tax liabilities, compliance requirements, and restrictions on usage or trading.
7. Market Manipulation and Ponzi Schemes: Cryptocurrency markets can be susceptible to manipulation and fraudulent schemes. Pump-and-dump schemes, where individuals artificially inflate the price of a cryptocurrency before selling their holdings, and Ponzi schemes promising high returns are risks within the cryptocurrency space.
8. Lack of Adoption and Usability: Cryptocurrencies still face challenges when it comes to mainstream adoption and usability. Limited acceptance as a payment method, scalability issues, complex user interfaces, and technological barriers can hinder the practical use and accessibility of cryptocurrencies.
It's important to note that while cryptocurrencies carry risks, advancements in technology, increased regulatory oversight, and the development of best practices and security measures are gradually improving the safety and stability of the cryptocurrency ecosystem. As with any financial instrument, users should exercise caution, conduct thorough research, and take appropriate security measures when engaging with cryptocurrencies.
Applications and Impact:
Blockchain's general purpose nature has sparked a multitude of applications across industries:
- Financial Services: Blockchain revolutionizes the financial sector with decentralized digital currencies, efficient cross-border payments, decentralized lending, and transparent trade finance.
- Supply Chain Management: Blockchain enhances supply chain transparency, traceability, and efficiency by providing an immutable record of every transaction and movement of goods, reducing fraud, counterfeiting, and supply chain disruptions.
- Healthcare: Blockchain ensures the secure and interoperable exchange of health records, improves data privacy and consent management, enables drug traceability, and facilitates clinical research and trials.
- Identity Management: Blockchain enables self-sovereign identity, empowering individuals to control their digital identities, securely share personal information, and streamline authentication processes.
- Energy and Sustainability: Blockchain facilitates peer-to-peer energy trading, grid management, carbon credit tracking, and transparency in renewable energy markets, enabling a more sustainable and decentralized energy ecosystem.
The future of blockchain technology is promising. As the technology matures, scalability challenges are addressed, and regulatory frameworks evolve, blockchain will continue to evolve as a powerful GPT. Collaboration among industry players, standardization efforts, and advancements in interoperability will further accelerate its adoption and impact.
Blockchain technology's classification as a general-purpose technology reflects its immense transformative potential and wide-ranging applicability. Its decentralized nature, trust-enhancing features, smart contracts, and interoperability lay the foundation for a new paradigm of secure, transparent, and efficient digital systems. As blockchain technology continues to evolve, its impact will transcend industries, drive innovation, and reshape the way we conduct business and interact in the digital age.
The Evolution, Enhancements, Efficiency, and Future of Hydrogen Fuel Cell Vehicles
Hydrogen Fuel Cell Vehicles and Electric Vehicles: Coexisting Solutions for Sustainable Transportation
Explore the coexistence of hydrogen fuel cell vehicles (FCVs) and electric vehicles (EVs) as complementary solutions in the transition to sustainable transportation. Discover how these technologies cater to different mobility needs, undergo technological advancements, and contribute to reducing greenhouse gas emissions.
In the quest for sustainable transportation solutions, hydrogen fuel cell vehicles (FCVs) have emerged as a promising alternative to traditional combustion engines and battery electric vehicles. Harnessing the power of hydrogen, these vehicles have undergone significant evolution, enhancements, and efficiency improvements over the years. This article explores the journey of hydrogen fuel cell vehicles, their advancements, efficiency gains, and prospects.
Evolution of Hydrogen Fuel Cell Vehicles: The concept of fuel cell technology dates back to the early 19th century, but it wasn't until the latter half of the 20th century that significant progress was made in developing practical fuel cell systems. In the 1960s, researchers began exploring the use of fuel cells in space missions. The subsequent decades saw incremental advancements, and by the 1990s, the automotive industry started exploring fuel cell technology for passenger vehicles.
The hydrogen fuel cell engine, also known as a hydrogen fuel cell vehicle (FCV), is an alternative powertrain technology used in automobiles. It utilizes hydrogen gas as the fuel source and converts it into electricity through an electrochemical process, which then powers an electric motor to propel the vehicle.
Here's a step-by-step breakdown of how a hydrogen fuel cell engine works:
1. Hydrogen Fuel: The fuel cell vehicle stores hydrogen gas in high-pressure tanks, usually located in the vehicle's chassis or trunk. Hydrogen can be produced through various methods such as steam methane reforming, electrolysis, or other renewable processes.
2. Fuel Cell Stack: The heart of the hydrogen fuel cell system is the fuel cell stack, which consists of multiple individual fuel cells. Each fuel cell contains an anode and a cathode separated by an electrolyte membrane.
3. Electrochemical Reaction: Hydrogen gas is fed to the anode side of the fuel cell stack, while oxygen from the air is supplied to the cathode side. At the anode, a catalyst causes the hydrogen molecules (H2) to split into protons (H+) and electrons (e-).
4. Proton Exchange Membrane: The electrolyte membrane allows protons to pass through while blocking electrons. This forces the electrons to flow through an external circuit, creating an electric current that can be used to power the vehicle's electric motor.
5. Oxygen Reaction: On the cathode side, oxygen from the air combines with electrons and protons that have traveled through the external circuit, along with the help of another catalyst. This forms water vapor (H2O), which is the only emission from a hydrogen fuel cell vehicle.
6. Powering the Electric Motor: The electric current generated by the fuel cell stack is then used to power an electric motor, which drives the vehicle's wheels and propels it forward. The fuel cell stack continuously produces electricity as long as there is a supply of hydrogen and oxygen.
7. Energy Storage: Hydrogen fuel cell vehicles typically incorporate energy storage systems, such as a small battery or a supercapacitor, to store excess energy generated during braking or deceleration. This stored energy can be used for additional power during acceleration or to provide electricity to the vehicle's auxiliary systems.
8. Efficiency and Environmental Benefits: Hydrogen fuel cell vehicles offer several advantages. They produce zero tailpipe emissions since the only by-product is water vapor. They have high energy efficiency since the fuel cell converts hydrogen to electricity directly, without the need for combustion. Furthermore, hydrogen can be produced from a variety of sources, including renewable energy, making it a potentially sustainable and low-carbon option for transportation.
It's worth noting that hydrogen fuel cell vehicles face challenges such as the limited availability of hydrogen refueling infrastructure and the cost of fuel cell technology. However, ongoing research and development efforts aim to address these issues and further improve the viability of hydrogen fuel cell engines for automobiles.
Enhancements in Technology: Over the years, extensive research and development efforts have propelled the enhancement of hydrogen fuel cell vehicles. Key areas of progress include:
1. Cost Reduction: The cost of fuel cell technology has significantly decreased due to advancements in materials, manufacturing processes, and economies of scale. This has made FCVs more viable for commercialization.
2. Efficiency Improvements: Researchers have made significant strides in improving the efficiency of fuel cell systems, enabling better energy conversion rates from hydrogen to electricity. These enhancements have increased the range and overall performance of FCVs.
3. Durability and Reliability: Improvements in the durability and reliability of fuel cell stacks have extended the lifespan of FCVs, making them more competitive with conventional vehicles.
4. Refuelling Infrastructure: The development of hydrogen refueling infrastructure has been a crucial focus for the expansion of FCVs. Although challenges remain, progress has been made, with hydrogen stations becoming more accessible in select regions.
Efficiency Gains and Environmental Benefits: Hydrogen fuel cell vehicles offer notable efficiency gains and environmental benefits:
1. Energy Efficiency: Fuel cell systems are more energy-efficient compared to internal combustion engines, as they directly convert chemical energy into electricity without the need for combustion. This results in higher efficiency and reduced energy wastage.
2. Zero Emissions: FCVs produce zero tailpipe emissions, with the only by-product being pure water vapor. This attribute makes them a significant contributor to reducing greenhouse gas emissions and combating air pollution.
3. Fast Refuelling and Extended Range: Unlike battery electric vehicles, hydrogen fuel cell vehicles can be refueled quickly, similar to traditional gasoline vehicles. Additionally, FCVs can offer an extended driving range, making them suitable for long-distance travel.
Future Prospects: The future of hydrogen fuel cell vehicles holds tremendous potential:
1. Infrastructure Expansion: Governments, private companies, and research institutions are investing in the development of hydrogen infrastructure, aiming to establish a robust refueling network. This expansion will help overcome a significant barrier to the widespread adoption of FCVs.
2. Technological Advancements: Ongoing research focuses on further enhancing the efficiency and performance of fuel cell systems. Innovations in catalyst materials, membrane technologies, and system design are anticipated to improve durability, reduce costs, and increase power density.
3. Renewable Hydrogen: The production of hydrogen from renewable sources, such as wind, solar, or hydroelectric power, is gaining traction. Renewable hydrogen offers the potential for an environmentally friendly and sustainable energy source for FCVs.
4. Industry Collaboration: Automakers, energy companies, and governments are increasingly collaborating to accelerate hydrogen fuel cell technology development and adoption. Such partnerships can foster innovation, standardization, and economies of scale, making FCVs more accessible and affordable.
The evolution of hydrogen fuel cell vehicles has witnessed remarkable progress in terms of technology, efficiency, and environmental impact. With continued enhancements, cost reductions, and infrastructure expansion, FCVs have the potential to play a significant role in the future of sustainable transportation. As we move toward a decarbonized future, hydrogen fuel cell vehicles could offer zero-emission mobility with long-range capabilities, contributing to a cleaner and greener transportation ecosystem.
The impact of green hydrogen in hydrogen fuel cell vehicles (FCVs) can be transformative for the transportation sector and the broader goal of achieving a sustainable, low-carbon future. Green hydrogen refers to hydrogen produced through electrolysis, using renewable energy sources such as solar, wind, or hydroelectric power. Here are the key impacts of green hydrogen in FCVs:
1. Carbon Neutrality: Green hydrogen offers the advantage of being produced without generating greenhouse gas emissions. As a result, when green hydrogen is used as a fuel source in FCVs, it ensures that the entire energy cycle, from production to consumption, is carbon neutral. This makes FCVs powered by green hydrogen a zero-emission transportation solution, contributing to mitigating climate change and reducing air pollution.
2. Renewable Energy Integration: The production of green hydrogen provides an avenue for integrating and storing excess renewable energy that would otherwise go to waste during periods of low demand. This helps in balancing the intermittent nature of renewable energy sources and enables the efficient utilization of renewable power. FCVs can play a crucial role in this energy system by utilizing green hydrogen as a clean energy carrier for transportation, thereby supporting the overall integration of renewable energy into the grid.
3. Energy Security and Independence: Green hydrogen production reduces reliance on fossil fuels for transportation. By promoting the adoption of FCVs fuelled by green hydrogen, countries can enhance their energy security and reduce dependence on imported oil. Domestic production of green hydrogen can be achieved using locally available renewable resources, providing a sustainable and indigenous energy solution.
4. Air Quality Improvement: Hydrogen fuel cell vehicles powered by green hydrogen have the potential to significantly improve air quality. Since FCVs produce only water vapor as a by-product, they eliminate tailpipe emissions of pollutants such as nitrogen oxides (NOx) and particulate matter (PM), which are major contributors to air pollution and adverse health effects. Deploying FCVs can contribute to cleaner and healthier urban environments.
5. Technological Advancements: The demand for green hydrogen in the transportation sector, including FCVs, can drive further advancements and cost reductions in hydrogen production, storage, and infrastructure. Increased investments and research in green hydrogen technologies can improve fuel cell system efficiency, durability, and affordability, making FCVs more accessible to a broader range of consumers.
6. Global Decarbonization Efforts: The widespread adoption of FCVs powered by green hydrogen aligns with global decarbonization objectives. As countries strive to reduce greenhouse gas emissions and transition to a clean energy future, green hydrogen can play a crucial role in decarbonizing the transportation sector. FCVs offer a viable alternative to fossil fuel-based vehicles, providing a pathway toward achieving ambitious climate targets.
The impact of green hydrogen in hydrogen fuel cell vehicles is substantial. It enables carbon-neutral transportation, supports renewable energy integration, improves air quality, enhances energy security, drives technological advancements, and contributes to global decarbonization efforts. By embracing green hydrogen, FCVs can offer a sustainable and efficient mobility solution that paves the way for a cleaner and greener future.
Hydrogen fuel cell vehicles (FCVs) and electric vehicles (EVs) are both part of the transition toward sustainable transportation. While FCVs and EVs share the goal of reducing greenhouse gas emissions and dependence on fossil fuels, they utilize different technologies. It is important to note that the adoption of one technology does not necessarily mean the demise of the other. Here's why:
1. Complementary Solutions: FCVs and EVs serve different use cases and have their strengths. FCVs, with their longer driving range and quick refueling capabilities, may be more suitable for long-distance travel and heavy-duty applications. On the other hand, EVs excel in urban environments and shorter commutes, benefiting from extensive charging infrastructure and rapidly improving battery technology. By offering diverse options, both technologies can coexist and cater to different consumer needs.
2. Market Demand and Consumer Preferences: The market for sustainable transportation is still growing, and consumer preferences vary. While some may opt for FCVs due to their range and refueling convenience, others may choose EVs for their simplicity, existing charging infrastructure, and familiarity. The market will likely accommodate both technologies, as they target different segments and serve different mobility requirements.
3. Technological Advancements: Both FCVs and EVs continue to undergo technological advancements, making them more efficient, cost-effective, and appealing to consumers. Battery technology for EVs is advancing rapidly, resulting in increased range, reduced charging times, and declining costs. Similarly, research and development efforts for fuel cell technology are driving efficiency improvements, durability enhancements, and cost reductions. Continued innovation will likely lead to further improvements in both technologies, expanding their respective market shares.
4. Infrastructure Development: The availability and expansion of refueling and charging infrastructure play a significant role in the adoption of both FCVs and EVs. While EV charging infrastructure has seen substantial growth, hydrogen refueling infrastructure for FCVs is still developing and faces challenges. However, efforts are underway to expand hydrogen refueling stations, making FCVs more accessible in regions with suitable infrastructure.
5. Environmental Considerations: Both FCVs and EVs contribute to reducing greenhouse gas emissions and improving air quality. The environmental benefits of each technology depend on factors such as the source of electricity or hydrogen production. EVs can leverage the increasing use of renewable energy sources, whereas FCVs can utilize green hydrogen produced from renewable energy, further enhancing their sustainability.
In conclusion, rather than competing directly, hydrogen fuel cell vehicles and electric vehicles are more likely to coexist and complement each other as part of a diverse and evolving sustainable transportation ecosystem. Consumer preferences, technological advancements, infrastructure development, and environmental considerations will collectively shape the market and determine the role each technology plays in the transition to a low-carbon future.
Snowflake: Revolutionizing Data Warehousing for the Future
In the rapidly evolving world of data analytics and storage, Snowflake has emerged as a game-changer. With its unique architecture, cloud-native approach, and innovative features, Snowflake has redefined how organizations store, analyze, and leverage data. This article delves into Snowflake's inception, evolution, progress, prospects, benefits, implementation approaches, best practices, and the organizations best suited to leverage its capabilities.
Overview: Snowflake is a cloud-based data warehousing platform designed to store, analyze, and process large volumes of structured and semi-structured data. It is known for its ability to handle massive data sets, support complex data models, and enable fast and scalable analytics.
Here are some key aspects and features of Snowflake:
1. Architecture: Snowflake follows a unique architecture called the multi-cluster shared data architecture. It separates compute resources from storage, allowing users to scale compute power independently of the data storage. This architecture enables elastic scalability, as additional compute resources can be added or removed as needed, providing high performance and concurrency.
2. Cloud-based: Snowflake operates entirely in the cloud, leveraging the infrastructure and services of popular cloud providers such as Amazon Web Services (AWS), Microsoft Azure, and Google Cloud Platform (GCP). This cloud-native approach eliminates the need for organizations to manage hardware or software infrastructure, providing flexibility and reducing operational overhead.
3. Data storage: Snowflake utilizes a columnar storage format that organizes data by columns rather than rows. This format improves query performance, compression, and storage efficiency. Snowflake automatically handles data partitioning, indexing, and optimization, enabling fast data retrieval and minimizing storage costs.
4. Elastic scalability: Snowflake enables users to scale their compute resources up or down to accommodate changing workloads. This scalability allows organizations to handle varying levels of data processing demands without manual intervention. It ensures efficient resource utilization and cost optimization.
5. Concurrency and performance: Snowflake is designed to support high levels of concurrency, allowing multiple users and applications to run queries simultaneously without contention. It achieves this by isolating the compute resources for each query, eliminating resource contention. Snowflake's architecture optimizes query execution through automatic query optimization, query acceleration techniques, and intelligent caching mechanisms.
6. Data sharing: Snowflake provides powerful data-sharing capabilities that allow organizations to securely and selectively share data with other Snowflake accounts. Data can be shared in real-time, enabling collaboration, analytics, and data monetization opportunities across different organizations or business units.
7. Security and governance: Snowflake incorporates robust security measures to protect data. It offers features such as end-to-end encryption, role-based access control (RBAC), data masking, and auditing. Snowflake also supports compliance with various industry regulations and standards, including GDPR, HIPAA, and PCI DSS.
8. Ecosystem integration: Snowflake integrates with popular business intelligence (BI) tools, data integration platforms, and programming languages. This integration facilitates seamless data ingestion, transformation, and analysis, allowing organizations to work with Snowflake using their preferred tools and workflows.
Snowflake provides a powerful and flexible data warehousing solution that offers scalability, performance, and ease of use in a cloud-based environment. Its architecture, combined with its features and capabilities, makes it well-suited for organizations dealing with large volumes of data and complex analytical workloads.
Inception and Evolution: Snowflake was founded in 2012 by a team of data warehousing experts with a vision to revolutionize the way organizations handle and analyze data. The founders aimed to address the limitations of traditional data warehousing solutions, such as scalability, performance bottlenecks, and high operational costs. With this goal in mind, they designed Snowflake's unique multi-cluster shared data architecture, separating computing and storage, and leveraging the power of the cloud.
Since its launch, Snowflake has witnessed rapid growth and adoption in the market. It gained traction among organizations seeking a modern, cloud-based data warehousing solution that could handle massive data sets and support complex analytics workloads. Snowflake's continuous innovation, strong ecosystem integration, and emphasis on security and governance have further propelled its evolution as a leading data warehousing platform.
Progress and Future Outlook: Snowflake's journey has been marked by significant milestones and advancements. The platform has consistently improved its performance, scalability, and ease of use. It has introduced features like automatic query optimization, data sharing, machine learning integration, and advanced security capabilities. Snowflake has also expanded its global footprint by forming partnerships with major cloud providers, such as AWS, Azure, and GCP, making it accessible to organizations worldwide.
Looking ahead, Snowflake is poised to continue its growth trajectory. The platform is expected to further enhance its capabilities in areas like data integration, data governance, and AI-driven analytics. As organizations increasingly recognize the value of data as a strategic asset, Snowflake is well-positioned to play a pivotal role in enabling data-driven decision-making and unlocking new business opportunities.
Benefits of Snowflake:
1. Scalability: Snowflake's elastic scalability allows organizations to scale compute resources up or down to match workload demands, ensuring optimal performance and cost efficiency.
2. Performance: Snowflake's architecture and optimizations enable high query performance, concurrent access, and rapid data retrieval, empowering users with fast and efficient analytics capabilities.
3. Cost Efficiency: By decoupling computing from storage and providing automatic data optimization, Snowflake minimizes infrastructure costs and storage requirements, delivering a cost-effective solution for data warehousing.
4. Data Sharing: Snowflake's data-sharing capabilities enable secure collaboration and monetization opportunities, facilitating seamless data exchange between organizations.
5. Security and Compliance: Snowflake incorporates robust security measures, end-to-end encryption, and compliance support, ensuring data confidentiality, integrity, and regulatory compliance.
Implementation Approach and Best Practices: Implementing Snowflake involves several key steps:
1. Assess Requirements: Define the use cases, data sources, and analytical needs to identify the optimal implementation approach.
2. Data Modeling: Design a logical and efficient data model that aligns with business objectives and facilitates easy data retrieval and analysis.
3. Data Integration: Implement data ingestion pipelines and integrate Snowflake with existing systems and tools for seamless data flow.
4. Query Optimization: Leverage Snowflake's optimization features and best practices to ensure optimal query performance and resource utilization.
5. Security and Governance: Implement strong access controls, encryption, and monitoring mechanisms to safeguard data and comply with regulatory requirements.
6. Training and Adoption: Provide training and support to users and stakeholders to ensure effective utilization of Snowflake's capabilities.
Organizational Suitability: Snowflake caters to organizations of varying sizes and industries, particularly those with:
1. Large and Complex Data Sets: Organizations dealing with massive volumes of structured and semi-structured data benefit from Snowflake's scalable architecture and optimized storage capabilities.
2. Analytical Workloads: Companies requiring advanced analytics, data exploration, and complex queries find Snowflake well-suited for their needs.
3. Cloud-Native Focus: Organizations leveraging cloud services and seeking a fully managed, cloud-based data warehousing solution find Snowflake highly compatible.
4. Collaborative Data Sharing: Industries that benefit from secure data sharing and collaboration, such as healthcare, finance, and retail, find Snowflake's data-sharing capabilities invaluable.
Snowflake has redefined the landscape of data warehousing with its innovative architecture, cloud-native approach, and advanced features. Its ability to handle massive data sets, provide elastic scalability, deliver high-performance analytics, and ensure security and compliance have made it a preferred choice for organizations across industries. As Snowflake continues to evolve and expand its capabilities, it promises to shape the future of data warehousing, enabling organizations to unlock valuable insights and gain a competitive edge in the data-driven era.
The Digital Immune System: Safeguarding the Digital Frontier
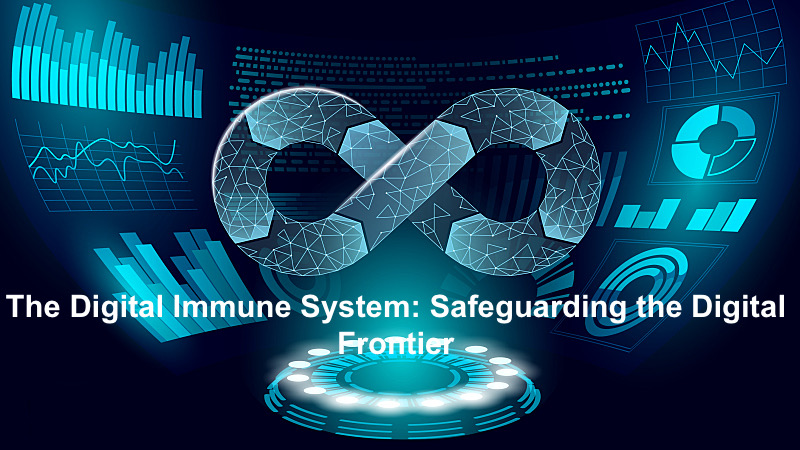
Discover how a Digital Immune System can protect your organization from cyber threats. Learn about its components, implementation approach, benefits, and best practices. Stay ahead of the evolving digital landscape with this comprehensive cybersecurity strategy. Defend against cyber threats with a Digital Immune System. Learn components, implementation, benefits, and best practices for robust cybersecurity.
In today's interconnected world, organizations face growing cyber threats that can compromise their valuable data and disrupt operations. The concept of a Digital Immune System has emerged as a proactive and holistic approach to cybersecurity, inspired by the human immune system. This article explores the components, implementation approach, benefits, best practices, and progression of Digital Immune Systems, offering insights into how organizations can fortify their defenses.
In the interconnected world, where digital threats continue to evolve and grow, organizations must fortify their cybersecurity defenses. The concept of a Digital Immune System has emerged as a proactive and holistic approach to protecting computer systems, networks, and data. Inspired by the human immune system, this comprehensive defense strategy combines advanced technologies, automated responses, and adaptive learning to defend against a wide array of cyber threats. In this article, we will delve into the components, implementation approach, benefits, best practices, and the progression of the Digital Immune System.
The term "Digital Immune System" refers to a concept inspired by the human immune system, but applied to the realm of digital technology and cybersecurity. Just as the human immune system defends the body against foreign invaders and diseases, a digital immune system is designed to protect computer systems, networks, and data from various threats and attacks.
A digital immune system is a holistic approach to cybersecurity that combines multiple layers of defense mechanisms and automated responses. It goes beyond traditional security measures like firewalls and antivirus software by employing advanced technologies such as machine learning, artificial intelligence, and behavioral analysis.
Here are some key aspects and features of a digital immune system:
1. Threat Detection: A digital immune system constantly monitors and analyzes network traffic, system logs, and user behavior to identify potential threats. It uses advanced algorithms and heuristics to detect patterns and anomalies that might indicate malicious activity.
2. Incident Response: When a threat is detected, the digital immune system responds swiftly and automatically. It can isolate affected systems, block suspicious network traffic, or initiate other protective measures to contain the threat and prevent further damage.
3. Adaptive Learning: A digital immune system can learn and adapt over time. It analyzes past incidents, attack patterns, and evolving threats to improve its detection capabilities. By continuously updating its knowledge base, it becomes more effective at identifying and mitigating emerging threats.
4. Collaboration: Digital immune systems often integrate with threat intelligence platforms and security information and event management (SIEM) systems. They can share information and collaborate with other security tools and systems to provide a comprehensive defense strategy.
5. Automated Remediation: In addition to detecting threats, a digital immune system can also take automated actions to remediate vulnerabilities and weaknesses in the system. This might include patching software vulnerabilities, closing security gaps, or updating security configurations.
6. Proactive Defense: Rather than solely relying on known threat signatures, a digital immune system employs proactive defense techniques. It uses behavioral analysis and anomaly detection to identify novel and zero-day threats that have not been previously identified.
7. Scalability: A digital immune system is designed to scale and adapt to different environments, from small networks to large enterprise infrastructures. It can handle high volumes of data and network traffic while maintaining its effectiveness.
The goal of a digital immune system is to provide a comprehensive and proactive defense against cyber threats, reducing the impact of attacks, and minimizing the response time. By combining advanced technologies and automated responses, it aims to create a resilient and secure digital ecosystem.
Components of a Digital Immune System:
1. Threat Detection: A Digital Immune System utilizes advanced algorithms, machine learning, and behavioral analysis to detect patterns and anomalies in network traffic, system logs, and user behavior. It continuously monitors and analyzes data to identify potential threats and emerging attack vectors.
2. Incident Response: Upon detecting a threat, the Digital Immune System responds swiftly and automatically. It can initiate various actions, such as isolating affected systems, blocking suspicious network traffic, or launching countermeasures to mitigate the threat and prevent further damage.
3. Adaptive Learning: A Digital Immune System learns and evolves. By analyzing past incidents, attack patterns, and emerging threats, it continuously updates its knowledge base to improve detection capabilities. This adaptive learning approach ensures that the system remains effective in an ever-changing threat landscape.
Implementation Approach:
Implementing a Digital Immune System involves several key steps:
1. System Architecture Design: Define the architecture and infrastructure required to support the Digital Immune System. This includes hardware, software, network components, and integration with existing security tools and systems.
2. Data Collection and Analysis: Set up mechanisms to collect and analyze relevant data, such as network traffic logs, system logs, and user behavior. This data serves as the foundation for threat detection and response.
3. Machine Learning and AI Integration: Integrate machine learning and artificial intelligence algorithms to enable advanced threat detection, anomaly detection, and predictive analysis. These technologies empower the system to identify and respond to threats in real-time.
4. Automation and Orchestration: Implement automated response mechanisms to minimize response time and reduce human intervention. Define predefined actions and workflows that the system can execute upon detecting a threat.
Benefits of a Digital Immune System:
1. Proactive Defense: By employing behavioral analysis and anomaly detection, a Digital Immune System can identify and mitigate emerging threats, including zero-day vulnerabilities, before they cause significant harm.
2. Scalability: The Digital Immune System is designed to scale and adapt to different environments, from small networks to large enterprise infrastructures. It can handle high volumes of data and network traffic without compromising its effectiveness.
3. Reduced Response Time: With automated response capabilities, the Digital Immune System can rapidly identify and neutralize threats, reducing the impact of attacks and minimizing potential damage.
4. Adaptive and Continuous Learning: The Digital Immune System continuously learns from past incidents and evolving threats, enhancing its detection capabilities and improving overall security posture.
Best Practices for Digital Immune System Implementation:
1. Comprehensive Threat Intelligence: Integrate threat intelligence feeds and collaborate with external security platforms to enhance threat detection and response.
2. Regular System Updates: Keep the Digital Immune System up to date with the latest security patches and software updates to ensure optimal protection against known vulnerabilities.
3. Employee Training and Awareness: Educate employees about cybersecurity best practices, such as strong password management, phishing awareness, and responsible online behavior. Human vigilance remains crucial in combating cyber threats.
4. Regular Auditing and Testing: Conduct regular audits and penetration testing to identify vulnerabilities in the system. This helps ensure that the Digital Immune System remains effective and resilient against new and evolving threats.
Progression of Digital Immune Systems:
Digital Immune Systems continue to evolve and progress in response to emerging threats and technological advancements. Some areas of progression include:
1. Integration with IoT and Cloud Security: As the Internet of Things (IoT) and cloud technologies become more prevalent, Digital Immune Systems are expanding their capabilities to protect these interconnected ecosystems.
2. Deeper Integration of AI and Machine Learning: Advances in AI and machine learning enable Digital Immune Systems to make more accurate predictions and automate complex decision-making processes.
3. Enhanced User Behavior Analytics: By analyzing user behavior patterns, Digital Immune Systems can better detect insider threats and unauthorized access attempts.
4. Collaboration and Information Sharing: Digital Immune Systems are increasingly sharing threat intelligence and collaborating with external security platforms to collectively combat cyber threats.
In an era where cyber threats pose significant risks to organizations, the Digital Immune System provides a robust and proactive defense mechanism. Combining advanced technologies, automated responses, and adaptive learning, it empowers organizations to detect, respond, and mitigate threats more effectively. With ongoing progress and best practices, the Digital Immune System continues to evolve, safeguarding the digital frontier in an increasingly interconnected world.
A Digital Immune System is a comprehensive cybersecurity strategy that plays a crucial role in an organization's overall cyber defense. It fits within an organization's cybersecurity framework as a proactive and holistic approach to protecting computer systems, networks, and data. It complements and enhances traditional security measures, such as firewalls and antivirus software, by incorporating advanced technologies like machine learning, artificial intelligence, and behavioral analysis.
The Digital Immune System operates at multiple levels within an organization's cybersecurity infrastructure:
1. Threat Detection: It actively monitors and analyzes network traffic, system logs, and user behavior to identify potential threats and anomalies.
2. Incident Response: Upon detecting a threat, the Digital Immune System triggers automated responses, such as isolating affected systems, blocking suspicious network traffic, or initiating countermeasures to mitigate the threat.
3. Adaptive Learning: The system continuously learns from past incidents and emerging threats, improving its detection capabilities and updating its knowledge base.
4. Collaboration: It integrates with other security tools and platforms, sharing information and collaborating to provide a comprehensive defense strategy.
By incorporating a Digital Immune System, organizations can enhance their cyber defense posture by proactively identifying and responding to threats, reducing response time, and leveraging advanced technologies to stay ahead of evolving cyber threats. It complements and strengthens the existing cybersecurity measures, providing a layered defense approach to protect critical assets and maintain operational continuity.
Current Status and Organization Size:
The concept of a Digital Immune System has gained significant traction in recent years as organizations recognize the need for more robust cybersecurity defenses. However, the adoption and implementation of Digital Immune Systems are still in varying stages across different industries and organization sizes.
Large Enterprises: Large enterprises, with their extensive networks and valuable assets, have been at the forefront of implementing Digital Immune Systems. These organizations often have dedicated cybersecurity teams and resources to deploy advanced technologies and build comprehensive defense strategies. They can invest in state-of-the-art tools, employ cybersecurity experts, and develop customized Digital Immune Systems tailored to their specific needs. Large enterprises are leveraging the benefits of Digital Immune Systems to protect their valuable data, customer information, and intellectual property.
Mid-sized Companies: Mid-sized companies, while not as resource-rich as large enterprises, are increasingly recognizing the importance of robust cybersecurity measures. Many mid-sized organizations are adopting Digital Immune Systems to strengthen their defenses against cyber threats. They might rely on a combination of in-house expertise and third-party cybersecurity solutions to implement and manage their Digital Immune Systems. These organizations are investing in technologies that provide threat detection, automated response, and adaptive learning capabilities.
Small Businesses: Small businesses often face resource constraints and may have limited cybersecurity expertise. However, with the growing prevalence of cyber threats, they are becoming more aware of the need to protect their digital assets. While the adoption of Digital Immune Systems in small businesses might be slower, there are cost-effective solutions available that provide essential security features. Small businesses are likely to leverage cloud-based security solutions and managed security service providers (MSSPs) to implement Digital Immune Systems within their budgetary constraints.
Emerging Trends and Future Outlook: The digital landscape is continually evolving, and so are the threats that organizations face. Digital Immune Systems are expected to adapt to these changes and embrace emerging trends to stay ahead of cybercriminals. Some notable trends and future developments include:
1. Artificial Intelligence and Machine Learning: The integration of AI and machine learning algorithms will continue to evolve, allowing Digital Immune Systems to better detect sophisticated threats and automate response mechanisms.
2. Zero-Trust Security: Organizations are moving towards a zero-trust security model, which assumes that no user or device should be inherently trusted. Digital Immune Systems will play a vital role in implementing and enforcing zero-trust principles, ensuring comprehensive security across the network.
3. Cloud and IoT Security: As cloud computing and IoT devices proliferate, Digital Immune Systems will expand their capabilities to provide robust security for these interconnected environments.
4. Collaboration and Threat Intelligence Sharing: Digital Immune Systems will increasingly collaborate with other security platforms and share threat intelligence to create a collective defense against evolving cyber threats.
While large enterprises have been at the forefront of implementing Digital Immune Systems, mid-sized companies, and small businesses are also recognizing their importance and gradually adopting these advanced cybersecurity measures. The future of Digital Immune Systems holds great potential as organizations of all sizes seek comprehensive defenses against an ever-changing threat landscape.
Wireless Electricity: Unleashing the Power of the Air
Wireless Electricity: From Inception to the Future - Revolutionizing Power Transfer
Discover the awe-inspiring world of wireless electricity, where power is transmitted without the need for wires or cables. From the groundbreaking principles of electromagnetic induction to the cutting-edge technologies of resonant coupling and beamforming, this article explores the evolution, applications, and prospects of wireless power transfer.
The advent of wireless electricity has brought about a paradigm shift in the way we think about powering our devices. By eliminating the need for physical connections, wireless power transfer has enabled greater convenience, flexibility, and portability. In this article, we will delve into the inception, evolution, and exciting prospects of wireless electricity. We will also explore the technologies that have made this revolutionary concept a reality.
Wireless electricity, also known as wireless power transfer or wireless energy transmission, refers to the transmission of electrical energy from a power source to an electrical device without the need for physical conductors such as wires or cables. This technology enables the transfer of electricity over short to moderate distances without the need for direct electrical connections.
The concept of wireless electricity has been explored and developed over the years using various techniques. One common method is electromagnetic induction, which involves the use of magnetic fields to transfer energy between two coils of wire. When an electric current flows through one coil, it creates a changing magnetic field around it. This changing magnetic field induces an electric current in the second coil, thus transferring energy wirelessly.
Another technique is resonant inductive coupling, which involves the use of resonant circuits to enhance the efficiency of power transfer. Resonant circuits are designed to have similar resonant frequencies, allowing them to exchange energy more efficiently. This method is often used in wireless charging systems for devices like smartphones or electric vehicles.
Wireless electricity can also be transmitted using technologies such as radio waves or microwaves. These methods involve converting electrical energy into electromagnetic waves, which can then be transmitted through the air and received by a device equipped with a compatible receiver. The receiver then converts the electromagnetic waves back into electrical energy, which can be used to power the device or charge its batteries.
One of the most well-known applications of wireless electricity is wireless charging for electronic devices. Wireless charging pads or mats use inductive coupling to transfer power to devices placed on them, eliminating the need for physical charging cables. This technology has become increasingly popular in recent years, and many smartphones, smartwatches, and other portable devices now support wireless charging.
It's worth noting that while wireless electricity offers convenience and flexibility, it still requires a power source to generate electrical energy. Whether it's a power outlet connected to the grid or a dedicated wireless charging station, electricity must be supplied to the system to enable wireless power transfer.
Overall, wireless electricity has the potential to revolutionize the way we power and charge our devices, offering greater convenience and eliminating the clutter of cables. Ongoing research and development in this field aim to improve efficiency, increase the range of wireless power transfer, and explore new applications for this technology.
Inception: The concept of wireless electricity traces its roots back to the late 19th century when visionary inventor Nikola Tesla first experimented with wireless power transmission. Tesla envisioned a world where electrical energy could be transmitted through the air, eliminating the need for power cables. His pioneering work on resonant inductive coupling laid the foundation for modern wireless power transfer.
Evolution: Over the years, advancements in technology and engineering have propelled the evolution of wireless electricity. Here are some key milestones:
1. Electromagnetic Induction: Tesla's experiments with electromagnetic induction led to the development of transformers, which are essential components in power transmission and distribution systems. This technology laid the groundwork for wireless energy transfer.
2. Radio Waves and Microwaves: In the early 20th century, the invention of radio and microwave technologies further expanded the possibilities of wireless power transmission. Radio waves and microwaves could carry electrical energy over longer distances, paving the way for wireless communication and remote powering applications.
3. Resonant Inductive Coupling: Resonant inductive coupling emerged as a significant breakthrough in wireless electricity. This technique utilizes resonant circuits to enhance power transfer efficiency. It is commonly employed in wireless charging systems for consumer devices such as smartphones and electric vehicles.
4. Wireless Charging: The introduction of wireless charging pads and mats revolutionized the way we recharge our devices. These systems use inductive coupling or resonant coupling to transfer power to devices placed on them, eliminating the need for physical cables. Wireless charging has become increasingly popular, with many devices incorporating this feature.
Prospects: The future of wireless electricity holds immense potential for further innovation and widespread adoption. Here are some exciting developments and technologies on the horizon:
1. Long-Range Wireless Power Transfer: Researchers are exploring ways to increase the distance over which wireless electricity can be transmitted. Technologies such as highly resonant wireless power transfer (HR-WPT) and beamforming techniques show promise in extending the range of wireless power transfer.
2. Radio Frequency (RF) Harvesting: RF harvesting involves capturing and converting ambient radio frequency signals into usable electrical energy. This technology could enable self-powered devices that draw energy from the surrounding environment, reducing the reliance on traditional power sources.
3. Over-the-Air Wireless Charging: Imagine a world where your devices charge automatically as you move around a room or an entire building. Over-the-air wireless charging aims to achieve precisely that. By utilizing advanced antenna arrays and beamforming, power can be transmitted to devices without the need for direct contact or alignment.
4. Internet of Things (IoT) and Smart Grids: Wireless electricity can play a pivotal role in powering the ever-expanding IoT ecosystem. By seamlessly integrating wireless charging capabilities into IoT devices, we can create a network of self-sustaining and wirelessly powered devices. Additionally, wireless electricity can contribute to the development of smart grids by enabling efficient and flexible energy distribution.
Wireless electricity, or wireless power transfer, relies on several key technologies to deliver electrical energy without the need for physical connections. Here are some of the prominent technologies used in achieving wireless electricity:
1. Electromagnetic Induction: Electromagnetic induction is a fundamental principle behind wireless power transfer. It involves the use of two coils of wire—an emitter and a receiver—placed in proximity to each other. When an alternating current (AC) flows through the emitter coil, it creates a changing magnetic field. This changing magnetic field induces an electric current in the receiver coil, thus transferring energy wirelessly.
2. Resonant Inductive Coupling: Resonant inductive coupling is a technique that enhances the efficiency of wireless power transfer. It involves the use of resonant circuits in the emitter and receiver coils. These circuits are designed to have similar resonant frequencies, allowing them to exchange energy more efficiently. By matching the resonant frequencies, power transfer efficiency can be significantly improved.
3. Radio Waves and Microwaves: Radio waves and microwaves are electromagnetic waves that can carry electrical energy over long distances. These waves are used in technologies such as radio broadcasting and wireless communication. By converting electrical energy into radio waves or microwaves, power can be transmitted through the air and received by a device equipped with a compatible receiver. The receiver then converts the electromagnetic waves back into electrical energy.
4. Beamforming: Beamforming is a technique used to direct a focused beam of energy toward a specific target. It involves using an array of antennas or transmitters to create constructive interference, which concentrates the energy in a particular direction. By precisely controlling the direction of the transmitted energy, beamforming enables more efficient and targeted wireless power transfer over longer distances.
5. Near-Field Communication (NFC): Near-field communication is a technology that allows for short-range wireless communication between devices. It relies on electromagnetic induction and operates over a distance of a few centimeters. NFC is commonly used in applications such as contactless payment systems and wireless charging pads, where the device needs to be near the charging source.
6. RF Harvesting: RF harvesting involves capturing and converting ambient radio frequency signals into usable electrical energy. This technology takes advantage of the abundance of wireless communication signals present in the environment, such as Wi-Fi, cellular signals, and broadcast transmissions. RF harvesting systems use antennas and rectifiers to capture and convert the radio frequency energy into direct current (DC) electricity.
These technologies, in combination or individually, enable wireless power transfer and have paved the way for applications such as wireless charging for consumer devices, wireless sensor networks, and future possibilities like long-range wireless power transmission and over-the-air charging. Ongoing research and development aim to improve the efficiency, range, and scalability of wireless electricity technologies to drive their adoption and integration into various industries.
Wireless electricity has come a long way since Nikola Tesla's pioneering experiments. The evolution of technologies such as electromagnetic induction, resonant inductive coupling, and wireless charging has paved the way for a future where power transfer is no longer constrained by physical connections. As research and development continue, we can anticipate even greater advancements in long-range wireless power transfer, RF harvesting, over-the-air charging, and their integration into IoT and smart grid systems. The future of wireless electricity is bright, promising a world where powering our devices is seamless, efficient, and wire-free.
The Hyper-Connected, Intelligent World: Unleashing the Potential of Connectivity and AI
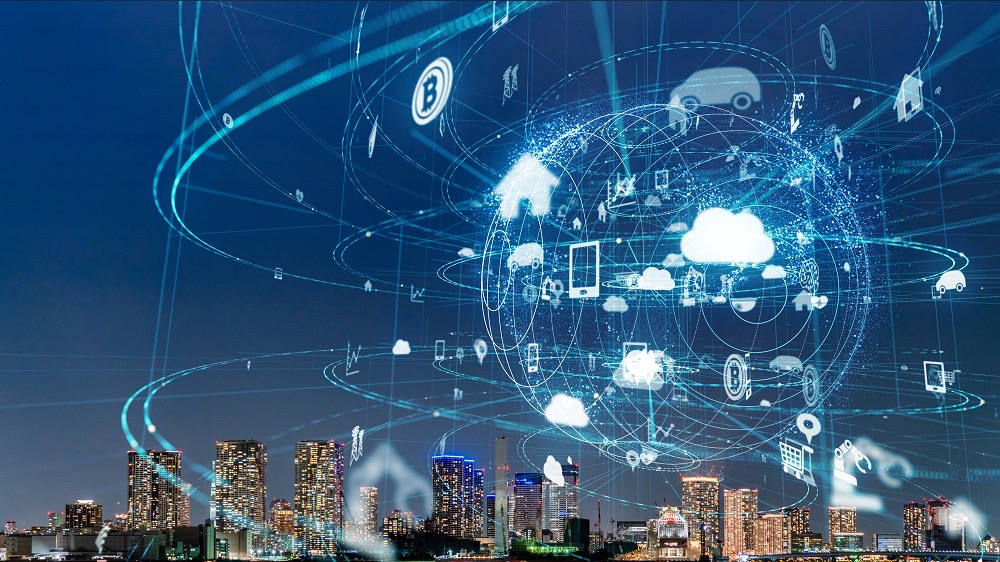
Discover the evolution, characteristics, prospects, implementation approaches, and best practices for embracing the hyper-connected, intelligent world. Explore how advanced technologies, pervasive connectivity, and artificial intelligence (AI) are transforming our society and shaping a future where seamless communication, personalized experiences, and intelligent decision-making thrive.
The hyper-connected, intelligent world refers to a future vision of our society where advanced technologies, pervasive connectivity, and artificial intelligence (AI) have significantly transformed the way we live, work, and interact with our surroundings. It envisions a highly interconnected ecosystem of devices, systems, and infrastructure that are seamlessly integrated and capable of intelligent decision-making.
Here are some key components and characteristics of the hyper-connected, intelligent world:
1. Internet of Things (IoT): The IoT is a network of physical devices, vehicles, appliances, and other objects embedded with sensors, software, and connectivity, enabling them to collect and exchange data. In a hyper-connected world, IoT devices are ubiquitous and play a vital role in connecting and gathering data from various sources.
2. Ubiquitous Connectivity: The hyper-connected world is characterized by pervasive connectivity, where high-speed internet access is available virtually everywhere. This allows seamless communication and data exchange between people, devices, and systems.
3. Artificial Intelligence (AI): AI technologies, such as machine learning, natural language processing, and computer vision, are integral to the hyper-connected world. AI enables machines and systems to analyze vast amounts of data, make intelligent decisions, and adapt to changing circumstances. It powers intelligent automation, predictive analytics, and personalized experiences.
4. Smart Cities: The hyper-connected world envisions the development of smart cities, where urban infrastructure is integrated with technology to enhance efficiency, sustainability, and quality of life. Smart city initiatives include intelligent transportation systems, energy management, optimized public services, and improved urban planning based on real-time data.
5. Intelligent Transportation: Transportation systems become smarter and more efficient in a hyper-connected world. Connected vehicles, infrastructure, and traffic management systems communicate and collaborate to optimize traffic flow, reduce congestion, improve safety, and enhance the overall transportation experience.
6. Personalized Experiences: With extensive data collection and AI-driven analysis, the hyper-connected world focuses on delivering personalized experiences across various domains. From personalized recommendations in e-commerce and entertainment to tailored healthcare treatments and education, technology adapts to individual preferences and needs.
7. Enhanced Connectivity and Communication: The hyper-connected world breaks down communication barriers through advanced connectivity solutions. People can interact seamlessly through various channels, including voice commands, augmented reality, virtual reality, and immersive technologies.
8. Intelligent Homes and Appliances: Homes are equipped with intelligent devices and appliances that can be controlled remotely and interconnected through a network. AI-powered assistants, voice recognition, and automation systems allow for seamless management of household tasks, energy consumption, and security.
9. Enhanced Healthcare: In a hyper-connected world, healthcare is revolutionized through connected medical devices, telemedicine, and AI-powered diagnostics. Patient monitoring, remote consultations, and predictive analytics enable more efficient and personalized healthcare delivery.
10. Data Security and Privacy: As our world becomes more interconnected and reliant on data, ensuring robust security and privacy measures becomes crucial. In the hyper-connected world, advanced encryption, authentication mechanisms, and regulations are in place to protect sensitive information and maintain trust in the digital ecosystem.
While the hyper-connected, intelligent world presents numerous opportunities and benefits, it also raises ethical, societal, and privacy concerns. It is essential to strike a balance between technological advancements and responsible implementation to harness the full potential of this future vision while ensuring a sustainable and inclusive society.
The Hyper-Connected, Intelligent World: Evolution, Characteristics, and Future
The hyper-connected, intelligent world represents a remarkable paradigm shift in our society, driven by advanced technologies, pervasive connectivity, and artificial intelligence (AI). This article explores the evolution, characteristics, prospects, implementation approaches, and best practices for realizing the potential of this transformative vision.
Evolution: The journey towards the hyper-connected, intelligent world can be traced back to the advent of the internet and the subsequent development of IoT devices. The proliferation of smartphones, coupled with the emergence of cloud computing and AI technologies, has accelerated this evolution. The interconnection of devices, systems, and infrastructure has enabled seamless data exchange and intelligent decision-making, leading us to the threshold of a new era.
Characteristics:
- Pervasive Connectivity: The hyper-connected world is characterized by ubiquitous high-speed internet access, enabling seamless communication and data exchange across various devices and systems.
- IoT Integration: The interconnection of billions of IoT devices forms the foundation of the hyper-connected world, allowing for the collection and analysis of massive amounts of data.
- Artificial Intelligence: AI technologies, such as machine learning, natural language processing, and computer vision, empower systems to process and interpret data, make intelligent decisions, and adapt to changing circumstances.
- Smart Systems: Intelligent transportation, energy management, healthcare, and other domains benefit from the integration of advanced technologies, creating efficient and sustainable systems.
- Personalized Experiences: Hyper-connectivity and AI enable the delivery of personalized services and experiences tailored to individual preferences and needs.
- Enhanced Communication: The hyper-connected world facilitates seamless communication through various channels, including voice commands, augmented reality, and virtual reality.
- Data Security and Privacy: As connectivity increases, robust security measures and privacy regulations are essential to protect sensitive information and maintain trust in the digital ecosystem.
Future Prospects: The future of the hyper-connected, intelligent world is boundless. Here are some potential areas of growth and impact:
- Smart Cities: Urban environments will embrace intelligent infrastructure, optimizing energy consumption, transportation, waste management, and public services.
- Healthcare Revolution: Connected medical devices, telemedicine, and AI-powered diagnostics will revolutionize healthcare delivery, improving access, efficiency, and patient outcomes.
- Autonomous Systems: Advancements in AI and connectivity will pave the way for autonomous vehicles, drones, and smart homes that anticipate and fulfill our needs.
- Industrial Transformation: Manufacturing processes will become more efficient through the integration of IoT, AI, and automation, leading to improved productivity and quality.
- Sustainable Solutions: The hyper-connected world can drive sustainability initiatives by optimizing resource consumption, reducing emissions, and enabling smarter energy grids.
Implementation Approach: To implement the hyper-connected, intelligent world effectively, several key approaches are crucial:
- Collaboration: Public-private partnerships and cross-industry collaborations are essential to leverage expertise, share resources, and overcome implementation challenges.
- Robust Infrastructure: A reliable and secure digital infrastructure, including high-speed connectivity, cloud computing, and edge computing capabilities, is fundamental for enabling the hyper-connected world.
- Data Governance: Establishing transparent and ethical data governance frameworks is necessary to ensure the responsible and secure handling of sensitive information.
- AI Ethics: Guidelines and regulations should be developed to address the ethical considerations associated with AI, including bias, privacy, and accountability.
- User-Centric Design: Prioritizing user experience and inclusivity in the development of hyper-connected systems ensures that technology benefits all segments of society.
- Continuous Learning and Adaptation: Embracing a culture of innovation and continuous learning will help organizations and individuals adapt to evolving technologies and harness their full potential.
Best Practices: To maximize the benefits and minimize the risks of the hyper-connected, intelligent world, the following best practices should be considered:
- Privacy by Design: Embed privacy and security considerations into the design and development of systems and applications from the outset.
- Data Protection: Implement robust data protection measures, including encryption, access controls, and anonymization techniques, to safeguard personal and sensitive information.
- Transparent Algorithms: Promote transparency in AI algorithms and decision-making processes to build trust and ensure accountability.
- Stakeholder Engagement: Engage diverse stakeholders, including policymakers, industry leaders, and citizens, in shaping the hyper-connected world to foster inclusivity and avoid unintended consequences.
- Continuous Security Updates: Regularly update and patch systems to address emerging security vulnerabilities and stay ahead of potential threats.
- Ethical AI Use: Develop and adhere to ethical AI guidelines, ensuring fairness, transparency, and accountability in AI-driven decision-making processes.
The hyper-connected, intelligent world represents a future where advanced technologies and interconnected systems empower individuals, organizations, and societies. By understanding its evolution, embracing its characteristics, adopting responsible implementation approaches, and adhering to best practices, we can navigate this transformative landscape, unlocking its full potential for a sustainable, inclusive, and intelligent future.
The Evolution, Current Status, and Future of Sustainable Tech in IT: Building a Greener Digital Future
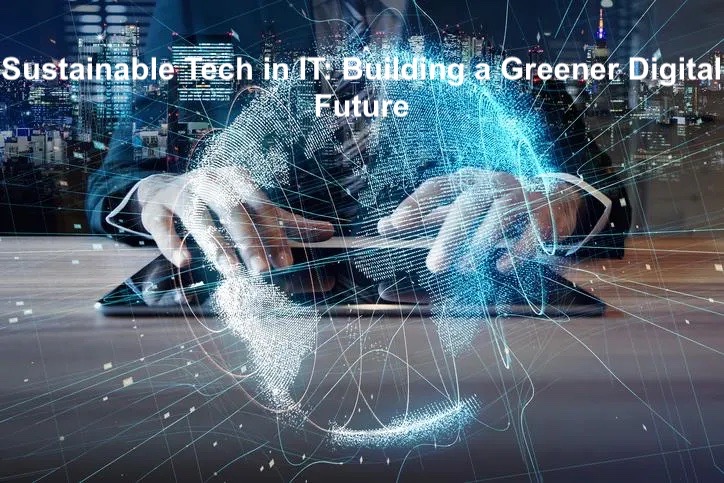
Sustainable tech, also known as clean tech or green tech, refers to the application of technology to create products, systems, and processes that minimize negative impacts on the environment and promote sustainable development. It aims to address pressing global challenges such as climate change, resource depletion, pollution, and loss of biodiversity.
Sustainable tech encompasses a wide range of technologies across various sectors, including energy, transportation, agriculture, construction, waste management, and more. Here are some examples of sustainable technologies:
1. Renewable Energy: Sustainable tech focuses on the development and deployment of renewable energy sources such as solar power, wind energy, hydropower, and geothermal energy. These sources generate electricity without depleting finite resources or emitting greenhouse gases.
2. Energy Efficiency: Sustainable tech aims to improve energy efficiency by developing technologies that reduce energy consumption in buildings, industrial processes, and appliances. This includes smart thermostats, LED lighting, efficient motors, and insulation materials.
3. Electric Vehicles (EVs): Sustainable tech promotes the adoption of electric vehicles as an alternative to fossil fuel-powered cars. EVs reduce greenhouse gas emissions, air pollution, and reliance on non-renewable energy sources when charged with renewable energy.
4. Green Building: Sustainable tech in construction focuses on designing and constructing energy-efficient buildings, using sustainable materials, and incorporating renewable energy systems. This includes features like solar panels, efficient insulation, rainwater harvesting, and smart building management systems.
5. Waste Management: Sustainable tech addresses the challenges of waste management through innovative solutions such as recycling technologies, waste-to-energy systems, composting methods, and improved waste sorting and disposal practices.
6. Precision Agriculture: Sustainable tech is transforming agriculture by incorporating digital tools, sensors, and data analytics to optimize crop production, reduce resource use, and minimize environmental impacts. This includes precision irrigation, drone monitoring, and smart farming systems.
7. Circular Economy: Sustainable tech promotes the concept of a circular economy, where resources are used efficiently, waste is minimized, and products are designed for reuse, repair, and recycling. Technologies such as material recycling, remanufacturing, and product lifecycle assessment play a crucial role in achieving a circular economy.
8. Water and Air Purification: Sustainable tech aims to develop efficient, cost-effective water and air purification solutions. This includes technologies like advanced filtration systems, water desalination, air pollution control systems, and indoor air quality monitoring.
Sustainable tech is focused on creating innovative solutions that balance economic growth with environmental protection and social well-being. It plays a crucial role in transitioning to a more sustainable and resilient future by reducing carbon emissions, conserving resources, and promoting a cleaner and healthier planet.
In recent years, the IT industry has recognized the need to address its environmental impact and embrace sustainable practices. The evolution of sustainable tech in IT has been driven by the growing awareness of climate change, the depletion of natural resources, and the desire to build a greener and more socially responsible digital future. This article explores the evolution, current status, and prospects of sustainable tech in IT, along with best practices, implementation approaches, and potential dangers.
Evolution of Sustainable Tech in IT: The journey of sustainable tech in IT can be traced back to the early 2000s when energy efficiency became a significant concern. The focus was primarily on reducing power consumption in hardware and optimizing data center operations. Over time, the concept expanded to encompass the entire IT lifecycle, including production, usage, and disposal.
The Current Status: Today, sustainable tech in IT has gained significant traction. Major players in the industry have embraced energy-efficient practices, renewable energy sourcing, and responsible e-waste management. Cloud computing and virtualization have become mainstream, enabling resource optimization and reduced hardware requirements. Additionally, organizations are increasingly leveraging data analytics and AI to drive sustainability initiatives.
Sustainable tech in the context of information technology (IT) refers to the application of environmentally friendly and socially responsible practices in the design, production, use, and disposal of IT products and services. It aims to minimize the environmental impact of the IT industry while maximizing the positive social and economic benefits. Here are some key aspects of sustainable tech in IT:
1. Energy Efficiency: Sustainable tech in IT focuses on reducing energy consumption in computing devices, data centers, and network infrastructure. This includes the development of energy-efficient hardware components, power management software, and virtualization technologies that optimize resource utilization.
2. Renewable Energy for Data Centers: Data centers, which are crucial for storing and processing vast amounts of digital information, consume significant amounts of energy. Sustainable tech promotes the use of renewable energy sources to power data centers, such as solar, wind, and hydroelectric power. It also encourages locating data centers in areas with access to renewable energy grids.
3. Cloud Computing and Virtualization: Cloud computing and virtualization technologies enable resource sharing and consolidation, reducing the need for physical infrastructure and energy consumption. By utilizing these technologies, organizations can optimize their IT infrastructure, reduce hardware requirements, and improve energy efficiency.
4. E-Waste Management: Sustainable tech in IT emphasizes responsible e-waste management. It encourages the recycling, refurbishment, and proper disposal of electronic devices to minimize the environmental impact of electronic waste. This includes implementing take-back programs, partnering with certified e-waste recyclers, and designing products with recycling in mind.
5. Green Software Development: Sustainable tech encourages the development of software that promotes energy efficiency and reduces resource consumption. This includes optimizing code for performance, minimizing data storage requirements, and designing applications that have a low environmental impact in terms of processing power and network bandwidth.
6. Sustainable Supply Chain: Sustainable tech involves considering the environmental and social impacts of the entire IT supply chain. This includes responsibly sourcing raw materials, reducing hazardous substances in manufacturing, and ensuring fair labor practices throughout the supply chain.
7. Telecommuting and Remote Work: Sustainable tech promotes remote work and telecommuting, reducing the need for daily commuting and associated carbon emissions. IT tools and platforms facilitate this, enabling virtual collaboration, video conferencing, and remote access to systems and data.
8. Data Analytics for Sustainability: Sustainable tech utilizes data analytics and AI to gain insights into resource consumption, environmental impact, and sustainability performance. By analyzing large datasets, organizations can identify areas for improvement, optimize processes, and make data-driven decisions that lead to more sustainable practices.
By implementing sustainable tech practices in IT, organizations can reduce their carbon footprint, minimize waste, optimize energy usage, and contribute to a more environmentally friendly and socially responsible IT industry. This, in turn, can help drive the transition to a sustainable and low-carbon economy.
Future of Sustainable Tech in IT: The future of sustainable tech in IT holds immense promise. Here are some key areas to watch:
1. Renewable Energy-Powered Data Centers: The shift toward renewable energy sources for powering data centers will continue, with a focus on solar, wind, and hydroelectric power. The integration of energy storage solutions will enable round-the-clock operations using clean energy.
2. Edge Computing and IoT: Edge computing, which processes data closer to the source, coupled with the Internet of Things (IoT), will enable more efficient and localized computing, reducing the need for large-scale data transfers and minimizing energy consumption.
3. Circular IT Economy: The concept of a circular economy will gain prominence, with IT companies designing products for durability, repairability, and recyclability. This will involve greater collaboration among manufacturers, consumers, and recyclers.
4. AI for Sustainable Decision-Making: Artificial intelligence and machine learning will play a crucial role in optimizing energy consumption, predicting maintenance needs, and driving sustainable decision-making across IT systems and infrastructure.
Best Practices and Implementation Approaches: To effectively implement sustainable tech in IT, organizations can follow these best practices:
1. Conduct Energy Audits: Start by assessing energy usage and identifying areas for improvement. Data centers, cooling systems, and hardware infrastructure should be prioritized.
2. Embrace Energy Efficiency: Optimize power management, utilize energy-efficient hardware, implement virtualization, and leverage software solutions that promote energy-saving practices.
3. Renewable Energy Adoption: Transition to renewable energy sources through on-site generation, power purchase agreements (PPAs), or renewable energy certificates (RECs). Collaborate with utility providers to ensure a clean energy supply.
4. Responsible E-Waste Management: Establish e-waste management policies, partner with certified recyclers, and implement take-back programs for proper disposal and recycling of electronic devices.
5. Sustainable Procurement: Consider environmental and social criteria when selecting IT vendors and products. Look for certifications such as Energy Star, EPEAT, and Responsible Minerals Initiative (RMI) compliance.
Dangers and Considerations: While sustainable tech in IT brings immense benefits, some potential dangers and considerations should be kept in mind:
1. Greenwashing: Organizations must be cautious of greenwashing, where companies exaggerate or misrepresent their sustainability efforts. Transparency and third-party certifications can help mitigate this risk.
2. Technological Advancement vs. E-Waste: Rapid technological advancements can lead to increased e-waste if not managed responsibly. Finding the right balance between innovation and sustainable product lifecycles is crucial.
3. Energy-Intensive Blockchain: Blockchain technology, although promising, is currently energy-intensive. Efforts are underway to develop energy-efficient consensus algorithms and blockchain scaling solutions.
Sustainable tech in IT has come a long way, transforming the industry's environmental impact and paving the way for a greener digital future. By embracing energy efficiency, renewable energy, responsible e-waste management, and circular economy principles, organizations can contribute to a sustainable and socially responsible IT ecosystem. The ongoing evolution, current status, and prospects highlight the importance of collaboration, innovation, and collective action to build a sustainable IT industry that drives positive change for the planet.